The Ocean's Potential Contributions to a Low-Carbon Economy: Analysis of Opportunities
Summary
Ocean Visions conducted an in-depth analysis to identify suitable opportunities for our Reduce program within the wide arena of potential ocean-based decarbonization pathways. The analysis researched over 20 ocean-based pathways to reduce greenhouse gas emissions in order to identify the highest-leverage opportunities that have not yet received sufficient investment of time, energy, and resources to reach their potential. The analysis included a review of published materials by initiatives such as the High-Level Panel for a Sustainable Ocean Economy paired with original analysis from Ocean Visions. Where available, our analysis used assessments of projected growth of these technologies within the next two decades. If not available, we used the median growth rates of ocean-based industries in their initial scale-up phases (e.g., offshore oil and gas, offshore wind, and aquaculture). Some of these pathways may have a higher theoretical potential, which could be unlocked by breakthroughs arising from more aggressive investment.
Our analysis looked at several parameters, including:
- CO₂-equivalent (CO₂e) mitigation potential: We looked at the potential for these pathways to reduce CO₂e emissions in the medium-term (2030-39) and in the long-term (2030-2050). CO₂e expresses the impact of different greenhouse gases in terms of the amount of CO₂ that would have the same global warming potential. Our analysis was based on available assessments of projected growth of these technologies where available, as well as the CO₂ e footprint of the goods or services that the pathway is replacing.
- Technology Readiness Level (TRL): The TRL scale (from 1-9) is used to enable assessment of the maturity of a particular technology and the consistent comparison of maturity between different types of technologies. Technologies range from those backed up by published research but untested (TRL 1) to those where complete systems have been successfully tested in real-world mission or market conditions (TRL 9). Technologies in the range of TRL 5-7 are particularly well suited for catalytic investment to enable their de-risking and scaling. Our analysis used existing sources where available.
- Market Size: This is an estimate of the size of the market that these technologies could command in 2030. Where available, we used existing market sizes and current growth rates from reputable sources to calculate 2030 market sizes.
See the complete table below of all decarbonization pathways in our analysis and how they ranked on key criteria. You can also download the table and review the underlying analysis and assumptions.

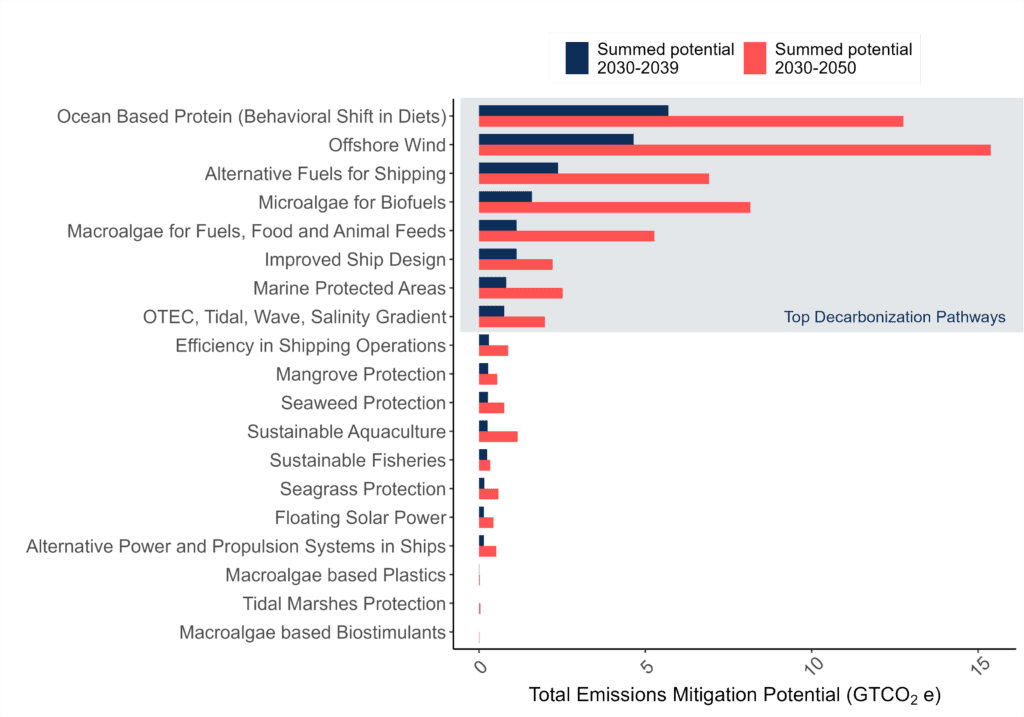
Our analysis indicates that eight different ocean-based decarbonization pathways may each have an impact of more than 1 billion tons (1 gigaton) CO₂e emissions reduction potential over 10 years. These include pathways that can decarbonize large parts of the world economy such as maritime transportation and food.
- Decarbonization efforts in the maritime sector are currently focused on alternative fuels, with a range of options being explored, including biofuels, e-methanol, e-ammonia, and green hydrogen. The long lifespans of ships, the difficulties associated with investing in new engines compatible with a range of fuel options, and the need for a new fuel bunkering infrastructure make this transition complex, but the transition will reduce emissions on the order of five gigatons CO₂e between 2030 and 2050.
- Similarly, a global transition away from land-based proteins to ocean-based proteins can have impacts exceeding 10 gigatons CO₂e between 2030 and 2050, mostly due to the reduction in emissions caused by lowered pressures on land-based agriculture.
Mature energy technologies, such as offshore wind, are also poised to scale and have significant impact despite the recent challenges in the sector such as reduced profitability due to rising interest rates and rising raw commodity prices as well as political headwinds in markets such as the United States.
Finally, emerging pathways like the use of marine algal biomass for fuels, food and animal feed, and a set of marine energy technologies have the potential to reach near gigaton-scale CO₂e in the period between 2030 and 2050.
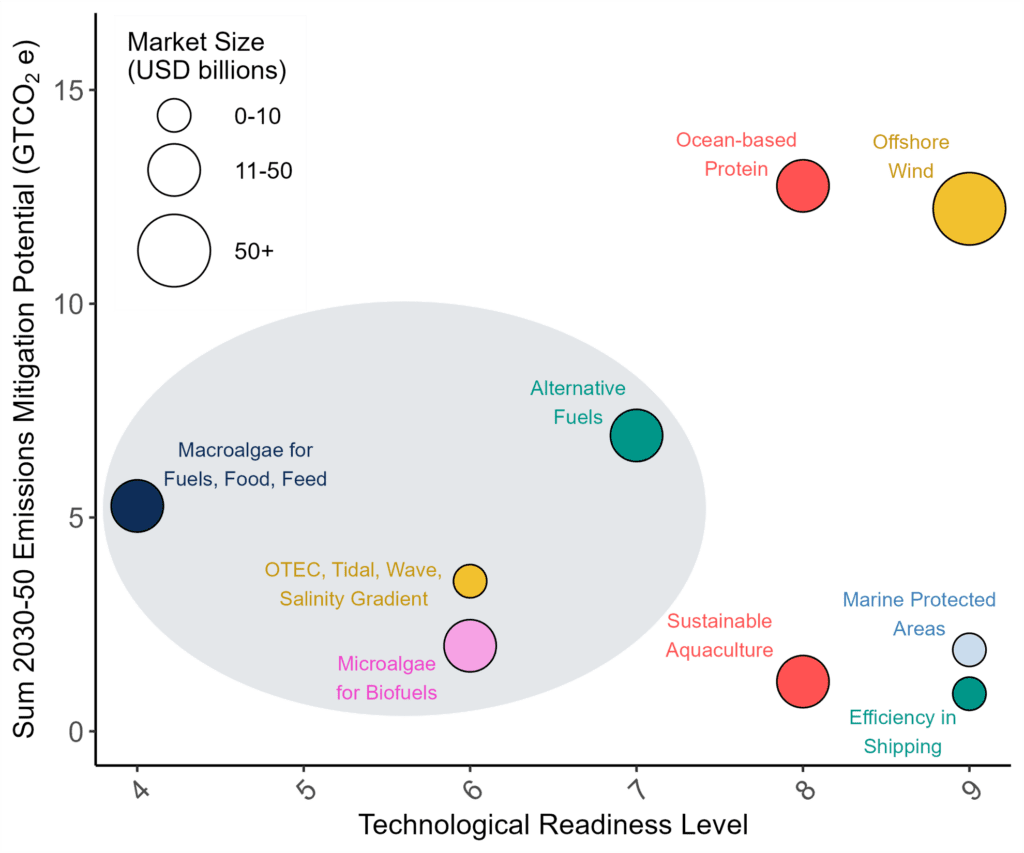
To illustrate this further:
- Offshore wind technologies have little scientific and technological risk and a large potential 2030 market. While floating offshore wind technology hasn’t been deployed at a commercial scale, the current need for advancing fixed and floating offshore wind is to build strong policy support.
- Ocean-based proteins can displace proteins derived from terrestrial sources and can sell into a large market quickly with limited need for technological development. The industry requires policy support and strategies to encourage large-scale behavioral shifts in diets to realize the enormous mitigation potential.
- Marine renewable energy solutions, biofuels, and other low-carbon intensity products from marine biomass and the development of alternative shipping fuels have slightly smaller but still significant mitigation potential and market size for 2030.
These solutions all fall within the TRL range of 5-7 and thus can benefit from catalytic investment in science and technology to accelerate these solutions and to help them reach their potential. To do this, we also need to mobilize strong links across academia, government, and the private sector.
Co-Benefits
Ocean-based decarbonization pathways provide a range of other benefits to communities within and beyond the coastlines, including jobs and economic development. Certain strategies for safeguarding ecosystems, such as mangroves and tidal marshes, may exhibit limited potential for mitigating emissions. However, these ecosystems provide critical benefits, including enhanced resilience to climate change impacts such as increased storm intensity. This underscores the necessity of investing in a diversified portfolio of climate solutions.
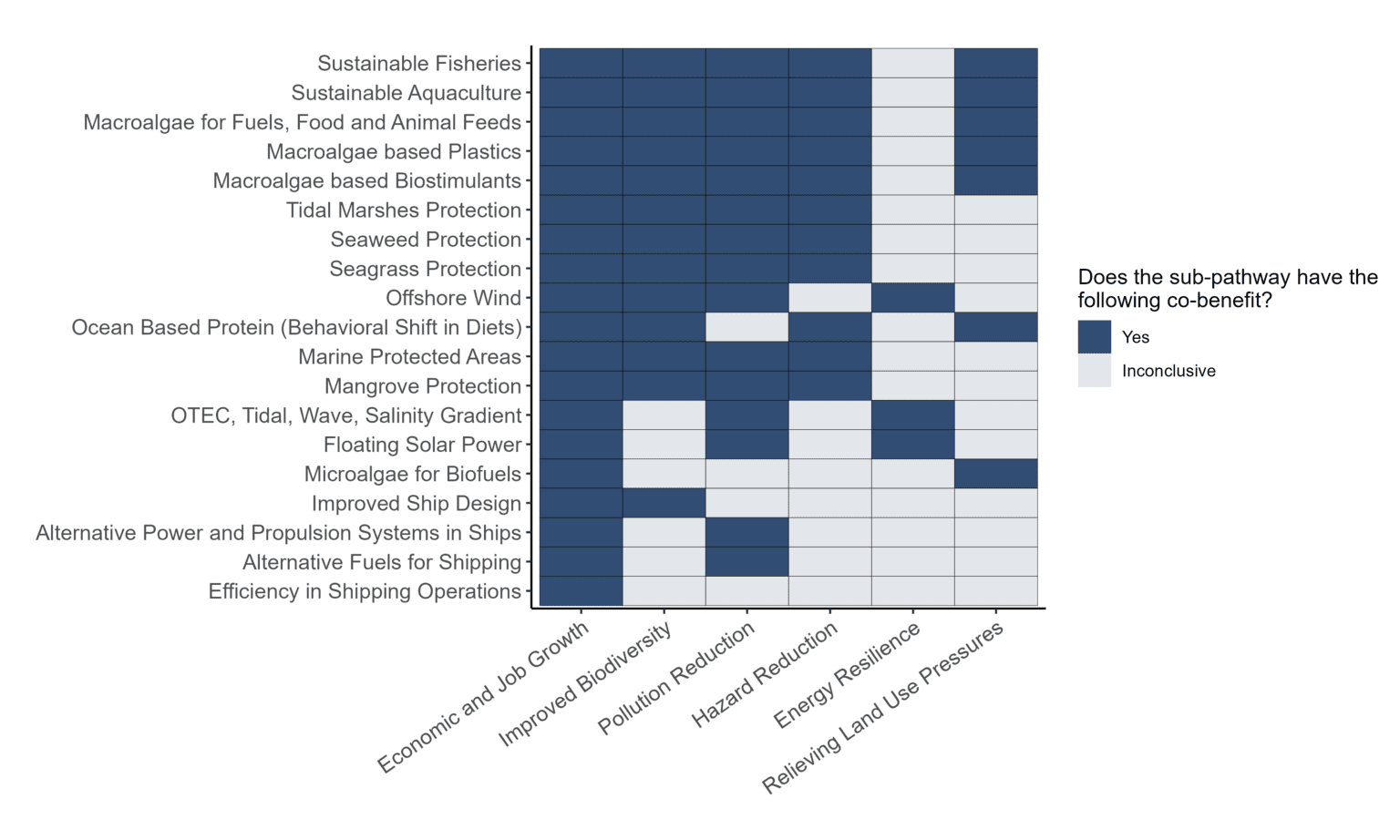
Conclusion
Our analysis was bolstered by a series of conversations with more than 40 experts working on different ocean-based decarbonization solutions. These experts brought diverse perspectives from several sectors, including academia, government, non-profits, and industry, and were able to add insights into the challenges and opportunities for each sub-pathway.
Based on this synthesis, we identified two areas of work focused on ‘Low-Carbon Seaweed-Based Products’ and ‘Marine Renewable Energy’ because we believe that these approaches can most benefit from our approach of supporting the development, design, and implementation of scalable yet underinvested solutions.
Ocean Visions Focal Areas
Low-Carbon Seaweed-Based Products: A Brief for Ocean Visions Reduce Program (click "+" to expand section)
Overview
- The use of macroalgae presents a significant opportunity for decarbonization by replacing high-emission products such as fossil fuels or, in some cases, reducing emissions in the lifecycle, such as with the use of Asparagopsis as a low-emission substitute cattle feed, which can reduce methane emissions.
- Techno-economic analysis suggests that replacing certain high-emission food products with seaweed-based alternatives, along with its use in fuels and animal feed, could potentially avoid one gigaton of annual emissions by 2050. This would necessitate a substantial increase in seaweed production, expanding the current farming area 30-fold with a 12 percent annual growth rate.
- Beyond macroalgae, there are opportunities related to microalgae-based alternatives to high-emissions products. Our initial program focus will be on the role of macroalgae in decarbonization.
Challenges to Widespread Adoption
Several challenges hinder the widespread adoption of macroalgae-based alternative products:
- Scientific and Engineering Challenges
- Research questions exist across the supply chain, from the environmental impact of large-scale cultivation, to the efficacy of biostimulants and the long-term health effects of using seaweed for cattle feed, for example.
- Seaweed biorefining (industrial process of converting biomass to energy and other valuable products such as chemicals) is in the early stages of commercial development, with questions remaining about product yield and maximum biomass utilization, as well as optimization for the processing lifecycle to reduce carbon emissions.
- Policy and Regulatory Challenges:
- Permitting and regulations for seaweed farms, particularly in the United States, can be a lengthy and complex process affecting both research and scale up efforts.
- There can be conflicting uses and “spatial squeeze” for near-coastal and offshore waters that can impede the siting of seaweed aquaculture.
- There is an undeveloped regulatory landscape on various uses of seaweed, particularly in the United States. For example, no federal food safety regulations exist for farmed seaweed, resulting in uncertainty around risks to farmers.
- Other Challenges:
- High costs of macroalgae production and processing, particularly in markets outside Asia, are a major challenge.
- There needs to be better connective links between supply and demand to build a reliable supply chain. Currently, there isn’t a clear demand signal from major industrial players that can enable planned scale up of seaweed supply.
- Climate change and the impact on seaweed ecosystems and ocean infrastructure pose a long-term risk to sustainability and resilience.
Key Players in the Macroalgae Area
- Non-Profits / For-Profit Companies:
- The most comprehensive list of NGOs and industry associations working on seaweed-based products can be found in this Phyconomy database.
- Academia:
- Numerous universities and research institutions that are part of the Ocean Visions network have research programs related to macroalgae cultivation.
- The Virtual Institute on Feedstocks of the Future initiative funded by Schmidt Sciences has sponsored a center called Sargassum Biorefinery (SaBRe), which includes scientists from Rutgers, Princeton University and Marine Biological Laboratory as co-leads to investigate the potential of using sargassum as an energy and food source.
- The International Bioeconomy Macroalgae Center (IBMC) at UC Berkeley will address the need for foundational knowledge, community engagement, and educational materials for consumers and businesses, among other initiatives, to build macroalgal-based bioeconomies.
Why We are Pursuing a Marine Algal Biomass Program
Ocean Visions possesses several strengths that align well with the challenges and opportunities in the marine biomass sector:
- Convening Power: Our strong network of partners across academia, industry, and government enables effective collaboration among diverse stakeholders. For example, Ocean Visions and the Monterey Bay Aquarium Research Institute convened a 20-person working group consisting of members across academia, government, and industry to improve the understanding of the effectiveness and consequences of sinking seaweed to the deep ocean for long-term carbon sequestration.
- Expertise in Roadmaps and Knowledge Products: We have a track record of developing comprehensive road maps and knowledge products that synthesize science advancements, identify research gaps, and prioritize actions. This aligns with the need for further research and development in the seaweed value chain.
- Support for Early-Stage Startups: The Launchpad program demonstrates our ability to connect startups with scientific advisors and facilitate research and development.
Specific Initiatives to Kick-Off the Program
Based on the challenges identified and Ocean Visions’ strengths, we identified the following area of work:
Road Mapping Exercise: We will organize and lead a collaborative process to identify, assess, and map out needed actions on the highest-value opportunities to scale up marine macroalgae-based replacements for conventional fuels, fertilizers, industrial feedstocks, and more. We will produce a web-based, interactive platform to publicize this work and to lay a foundation for future work, which will be focused on supporting RD&D, policy work, and broad field-building to move macroalgae alternatives to market.
Expected Outcomes
The road map will identify a set of tractable first-order priorities needed to advance the state of knowledge around a diverse array of algae-based technologies that will be able to contribute to global decarbonization. We will also identify parties working on these priorities and support the most promising efforts to advance development, and ultimately deployment, of the most promising technologies.
Marine Renewable Energy: A Brief for Ocean Visions Reduce Program (click "+" to expand section)
Overview
The ocean represents a vast and largely untapped source of renewable energy. Marine Renewable Energy (MRE) technologies, including wave, current (which includes tidal and river energy), Ocean Thermal Energy Conversion (OTEC), and osmotic energy (salinity gradient) could significantly contribute to global decarbonization efforts. The energy captured can be used for all different kinds of useful work, including electricity generation, water desalination, and water pumping. Analysis by Ocean Visions suggests that MRE could contribute a little under 100 million tonnes CO₂e of reduced emissions per year in the 2030s and beyond. Equally critically, MRE could help enable the scaling of industries, such as aquaculture and marine carbon dioxide removal (mCDR) further offshore, further amplifying its climate mitigation impact.
Definitions
Wave Energy: Wave energy is the transport and capture of energy by ocean surface waves.
Current Energy: Current energy can be captured from ocean currents or rivers. Ocean current energy technologies capture the energy from the relatively constant flow of ocean currents, which are driven by several factors, including wind, bathymetry, and the rotation of the Earth, as well as water temperature, density, and salinity.
Tidal energy: Tidal energy captures the energy from flow induced by the rise and fall of tides, which is driven by the moon and sun’s gravitational influence on the earth’s oceans.
Ocean Thermal Energy Conversion: Ocean thermal energy conversion (OTEC) is a process or technology for producing energy by harnessing the temperature differences (thermal gradients) between ocean surface waters and deep ocean waters.
Osmotic Energy or Salinity Gradient: Salinity gradient power is the energy created from the difference in salt concentration between two fluids.
MRE is important for several reasons including:
- Decarbonization: MRE can contribute to reducing societal greenhouse gas emissions by providing a clean alternative to fossil fuels.
- Energy Security: MRE can enhance energy security by diversifying energy sources and reducing dependence on imported fuels.
- Grid Resilience: Marine energy’s predictability can help support resilience objectives and local loads in locations that have poor renewable alternatives.
- Serving Remote Communities: MRE can provide access to clean and reliable energy for remote communities and islands with limited grid access.
- Powering Remote Operations: MRE can provide off-grid energy supply to distributed marine users, including potentially marine CDR.
- Job Creation: Development of the MRE sector has the potential to create new jobs and stimulate economic growth.
Challenges to Widespread Adoption
Several significant challenges hinder widespread adoption of MRE as part of a portfolio of renewable energy sources:
- Scientific and Engineering Challenges
- Most forms of MRE are still in early stages of technology validation. For example, several wave technology technologies are still being tested and most of them have Technology Readiness Levels (TRLs) between 5 and 7, indicating further research and development to reach commercial viability. OTEC technology has been tested and demonstrated for several years in real-world settings. However, high capital costs have resulted in the development of only a handful of projects.
- MRE devices must operate reliably in challenging ocean conditions, including strong waves, currents, and corrosive saltwater environments. Ensuring the long-term survivability of these devices is crucial for their commercial success. Reducing costs requires significant advancements in reliability for sub-components used in these devices.
- There are potential environmental impacts associated with MRE deployment. These include impacts on marine life, habitats, and oceanographic systems (causing changes to water circulation, wave heights, and current speeds). While several risks to habitats and oceanographic systems like noise or electromagnetic fields have been retired (considered to be understood and managed) for small arrays of MRE devices, entanglement, and collision risks and risks from larger arrays need to be studied further. Finally, a thorough understanding and translation of these impacts to key stakeholders, alongside the development of effective mitigation strategies, is essential for responsible MRE development.
- Policy and Regulatory Challenges:
- The process of obtaining permits for MRE projects is often complex, time-consuming, and inconsistent across jurisdictions. Projects can take many years to receive necessary approvals, creating significant uncertainty for developers and hindering investment.
- Integrating MRE into existing electricity grids presents technical challenges. The variable nature of wave and tidal energy requires sophisticated grid management strategies to ensure stability and reliability.
- Effective stakeholder engagement is essential for the successful development of MRE projects, but approaches vary widely. Building consensus among diverse stakeholders, including local communities, fishing industries, and environmental groups, requires carefully tailored engagement strategies.
- Other Challenges
- As a result of being nascent technologies, MRE technologies are currently more expensive than more established renewable energy sources, such as solar and wind power. For comparison, Levelized Cost of Energy (LCOE) for utility-scale solar projects is $29-$92 $/MWh, while forecasted LCOE for the first commercial-scale project was in the range of $120‒470/MWh for wave energy and $130‒280 $/MWh for tidal energy. This high cost is a major obstacle to MRE technologies attracting investment and competing in the energy market. Reducing costs will require identifying applications and geographies where MRE technologies have other advantages (for example, complementary generation patterns to wind and solar) or as in the case of OTEC, finding other uses for nutrition-dense cold water such as air-conditioning or as a resource to support fisheries.
- Limited public understanding of MRE technologies can lead to misconceptions about their benefits and potential impacts. This can hinder public acceptance and support for MRE projects.
- Investment, particularly in the United States (U.S.), is largely from the public sector. Political change can impact funding environments even though the U.S. funding for MRE has typically been bipartisan.
- The availability of pre-commercial test sites is limited, making it difficult for developers to test and validate their technologies in real-world conditions. This lack of testing infrastructure slows down the pace of innovation and commercialization. A number of initiatives have stepped up to fill this void. This includes TEAMER, Pac Wave, and the European Marine Energy Centre.
Key Organizations in Marine Renewable Energy
The marine energy sector involves a range of organizations, including:
- Government Agencies: The U.S. Department of Energy (DOE) Water Power Technologies Office (WPTO) is a key funder and works with “national laboratories, academic institutions, and companies across the country to advance technologies to harness hydropower and marine energy resources”. ARPA-E has funded marine renewable energy through its SHARKS program. Other potential U.S. government partners include the Department of Defense, Navy, and the National Oceanic and Atmospheric Administration (NOAA).
- Industry & Industry Associations: The Marine Energy Council (MEC) , under the National Hydropower Association, is a U.S. trade association for marine energy and advocates for the industry. Ocean Energy Europe includes Europe’s leading utilities, industrialists and research institutes, representing the interests of Europe’s ocean energy sector. Both parties’ websites have directories of industry members.
- National Laboratories: The National Renewable Energy Laboratory (NREL) and Pacific Northwest National Laboratory (PNNL) conduct research and development on marine energy technologies.
- Universities: Several universities are actively involved in marine energy research. The University Marine Energy Research Community (UMERC) fosters collaboration within the U.S. marine energy research community.
How Can Ocean Visions Advance Marine Renewable Energy?
Ocean Visions sees a number of opportunities to leverage our strengths and apply them in ways that can make a meaningful impact in the MRE sector.
- Support for early-stage innovation: Ocean Visions has a proven track record of supporting early innovators and catalyzing expert support to help them advance and optimize high-impact solutions. The MRE sector is still in its early stages of development, particularly in areas like wave energy and OTEC, and would benefit greatly from Ocean Visions’ capacity to help start-ups accelerate innovation.
- Fostering synergy with mCDR : Finding suitable demand for MRE applications will be an important element in moving them forward. Ocean Visions does significant work with mCDR start-ups and companies and believes MRE applications could provide a sustainable and reliable power source for some of these technologies. This would be a win-win and allow for both mCDR and MRE to grow and expand in a synergistic way. However, it’s worth noting that many mCDR companies are not yet venturing offshore as their immediate focus is on more established renewable energy sources.
Specific Initiatives to Kick-Off the Program
To launch an MRE program, we plan the following areas of work:
Open a Launchpad cohort to support early-stage companies advancing the use of MRE by providing them scientific, technical and policy advice from the Ocean Visions Network. Advisors drawn from the Ocean Visions Network and external experts will provide:
- Scientific and engineering mentorship
- Environmental impact assessment guidance
- Regulatory and permitting insights
- Market readiness and scale-up strategy support
- Communication and community engagement guidance
Expected Outcomes
By the end of the program, participants will have:
- Enhanced their technical and environmental assessments
- Advanced their field trials with expert input
- Strengthened their commercialization readiness
Resources
- Review the in-depth Ocean Visions Analysis of 24 sub pathways